Optical Network Research Team
Group Outline and Primary Goal
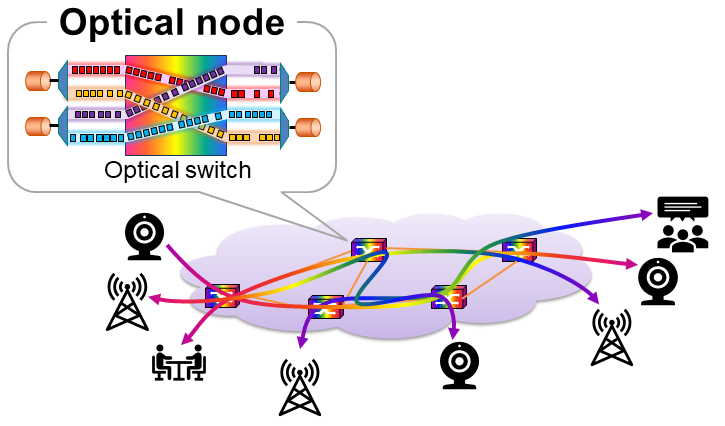
Figure 1: Dynamic optical-path network
Novel ICT systems such as “Continuum computing infrastructure” requires high-capacity and low-latency data communication infrastructure. Therefore, they will be built up based on advanced optical networks.
Optical Network Research Team is promoting research and development aiming for “Dynamic Optical Path Network”, where end-users are directly connected by optical fiber links and the paths of optical signals can be dynamically switched according to requests by the users. Such a configuration realizes ultra-high-capacity and low-latency networks with extremely low energy consumption. The goal of our research is to realize a network with fully automated operation based on virtualization technologies, enabling easy operation for everyone regardless of existence of the physical layer, and flexible connectivity with any places. We investigate key technologies such as new network architectures using optical switches, virtualized and automated control schemes of optical networks, and optical fiber transmission with advanced signal processing techniques.
Key Themes of Research
(1) Virtualization, control, and resource management technologies for future optical networks
In conventional optical communication network systems, the systems have been designed based on "all-in-one" equipment including all the functionalities (e.g., transponders, optical amplifiers, optical fiber spans). Disaggregation technologies in optical networks have recently been developed with the aim of introducing optical transport equipment into datacenter networks having requirements different from those of telecom networks or installing various optical equipment more flexibly within telecom networks. Disaggregation approaches decouple transport equipment, sub-systems, and optical devices so that customization and optimization of node structure assemblies can be realized more precisely.
Though the disaggregation approach can expand the flexibility of optical network systems, the control or management required in the software-defined networking (SDN) controllers or the network resource managers (NRMs) can become complicated because the disaggregation approach has to handle a larger number and higher variations of optical components. To resolve this issue, we have developed a modeling method which can handle optical functional blocks composing optical nodes and can be processed in an automated manner. Figure 2 outlines the developed functional block-based disaggregation model (FBD model). In conventional modeling methods, an optical node was modeled as one black box (which looks like the network-level model in Fig. 2). On the other hand, our developed FBD model can specify the optical node structures in detail as shown in Fig. 2. That is, individual optical components, such as WSSs and EDFAs, are first modeled (component model in Fig. 2); then an optical node structure is defined with the individually modeled components and optical fibers connecting the component ports (node-level model in Fig. 2), and then a whole network is built based on precisely defined optical nodes (network-level model in Fig. 2). Furthermore, a distinctive feature of the FBD model is that the optical paths between any two points on the network can be universally computed using the integer linear programming (ILP) method. In the FBD model description, the switching functionalities (i.e., constraints such as spectrum availability and input/output connectivity) of the individual optical components are accurately and mathematically defined in a machine-readable format using ILP formulation methods, which can be computed directly by any ILP solver. By computing the ILP formula, the switching functionalities for whole nodes or networks can be analyzed using automated processes. The automation realized by the FBD model will be a key to resolve the complexity required for SDN controllers or NRMs due to the disaggregation approach.
So far, we have demonstrated control and resource management system based on the FBD model implemented on a real hardware testbed [link], swift optical physical layer topology update enabled by the automated processings based on the FBD model [link], and evaluation of the computation time scalability of an FBD model based path computation prototype system [link]. Furtheremore, an open-source tool for generating an FBD model-based topology description has been published [link].
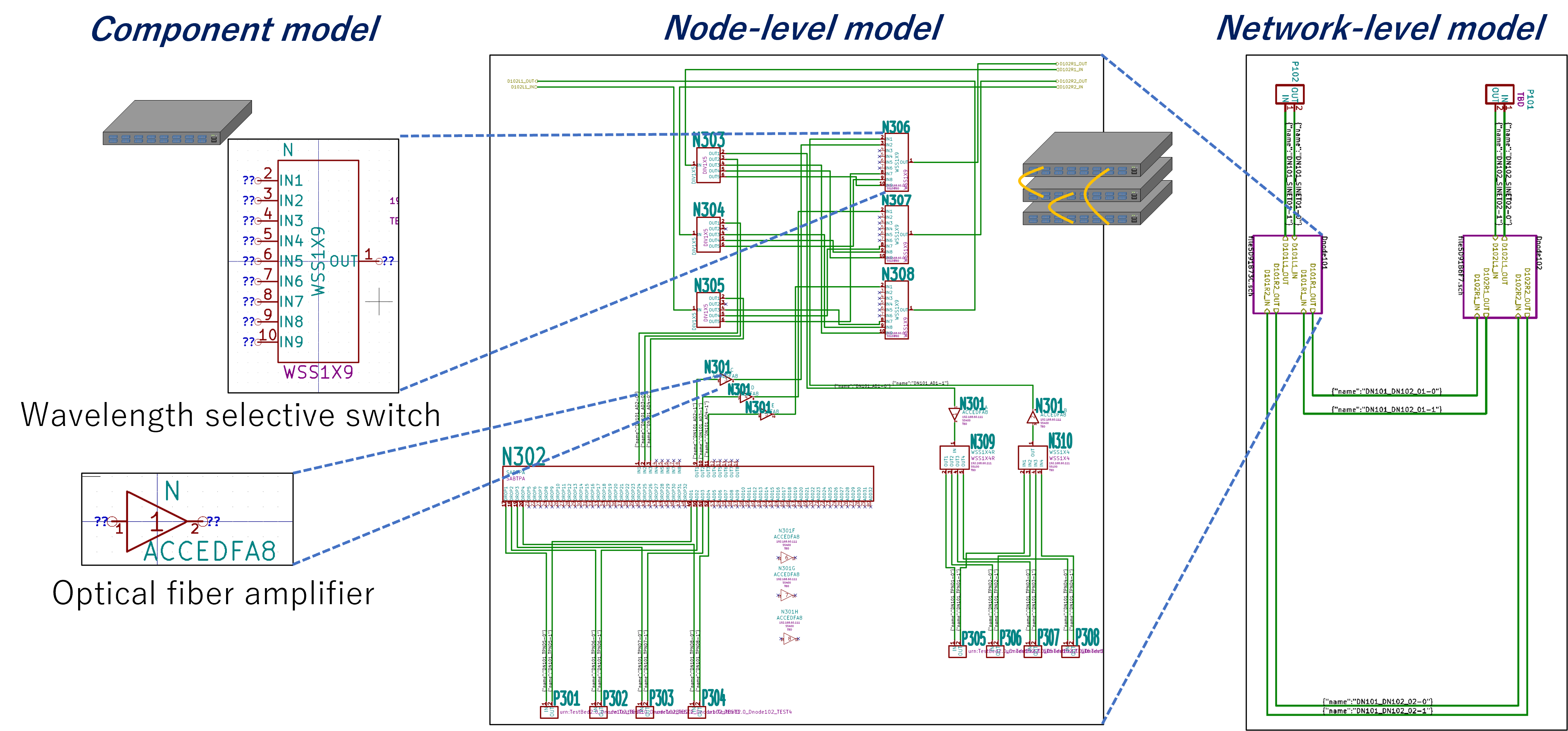
Figure 2: Schematic diagram of Functional Block-based Disaggregation model
(2) Large-scale and fast optical circuit switch systems for datacenter networks and next generation computing applications
Future intra-datacenter networks will confront the bandwidth crunch and power consumption limitation of electrical packet switches. A solution is the introduction of optical circuit switches. We investigate large-scale and fast optical circuit switch systems to meet some requirements (Port count > 1,000, Switching speed < a few microsecond) in datacenters. Figures 3 (a) and (b) show examples of large-scale and fast optical circuit switch systems. One is configured by a combination of wavelength routing and space switches, another is a cascaded configuration of silicon photonics matrix switches. For details, please refer to journal articles (link1, link2) and a press release.
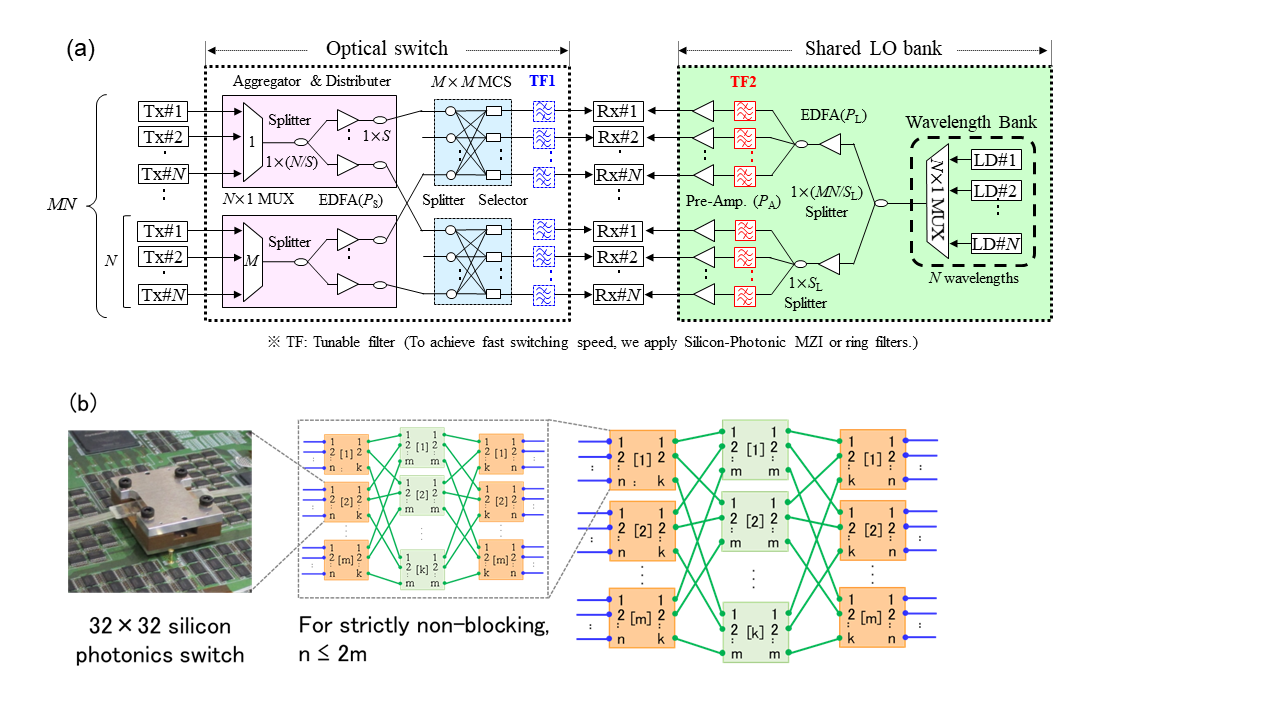
Figure 3: Examples of large-scale and fast optical circuit switch system
(3) Compensation of nonlinear waveform distortion of optical signals using digital signal processing with machine learning technique
High-capacity, wavelength-division-multiplexed (WDM) optical signals suffer from waveform distortion due to fiber nonlinearity after long-distance transmission. This limits the performance of optical transmission systems such as distance or information capacity, and how to overcome this limitation is extensively studied all over the world. We have been working on this research topic using either digital or optical signal processing techniques.
Figure 4 shows a block diagram to implement an algorithm for nonlinearity compensation, where back propagation of optical signals in transmission fibers is calculated by alternate linear and nonlinear segments in receiver-side digital signal processing. In nonlinear segments, cross-phase modulation (XPM) between WDM channels as well as self-phase modulation (SPM) which is an intra-channel nonlinear effect are considered for calculation to compensate for the nonlinear phase shift. We proposed a machine-learning technique to optimize the parameters used in the calculation and confirmed an improved performance of nonlinearity compensation through an optical transmission experiment. For details, please refer to a journal article.
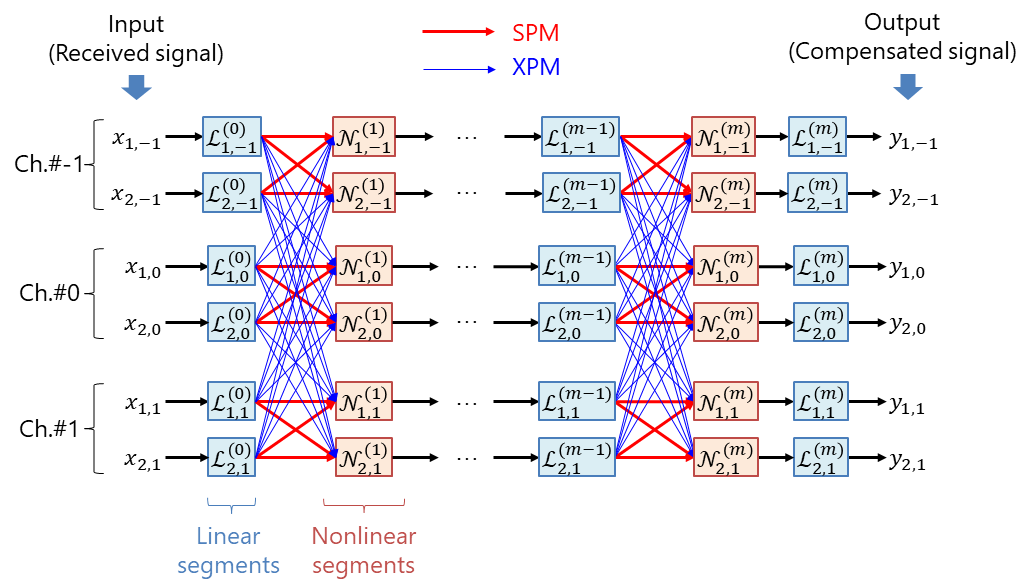
Figure 4: Block diagram to implement fiber nonlinearity compensation based on receiver-side digital signal processing.
Contact:

Our Technologies and Equipment
Design and operation of a dynamic optical path network test bed with a 90Tbps maximum capacity and 6kW power consumption
Optical network virtualization and controlling techniques
Evaluation of large-scale optical switch system using optical waveguide devices
Digital coherent transmission by advanced modulation/signal processing
Optical signal processing based on nonlinear fiber optics
Articles
- K. Ishii, S. Xu, N. Yoshikane, A. Takefusa, T. Tsuritani, Y. Awaji, and S. Namiki, "Automatic Mapping Between Real Hardware Composition and ROADM Model for Agile Node Updates," in J. Lightwave Technol., vol. 39, no. 3, pp. 821-832 (2021).
https://doi.org/10.1109/JLT.2020.3048424 - K. Ishii, A. Takefusa, S. Namiki and T. Kudoh, "Optical Network Resource Management Supporting Physical Layer Reconfiguration," J. Lightwave Technol., vol. 37, no. 21, pp. 5442-5454, (2019).
https://doi.org/10.1109/JLT.2019.2935789 - K. Ishii and S. Namiki, "Scalability of integer linear programming path computation for functional block-based disaggregation supporting a flexible grid mechanism [Invited]," in J. Opt. Commun. Netw., vol. 14, no. 2, pp. A134-A142 (2022).
https://doi.org/10.1364/JOCN.439820 - K. Ishii, R. Matsumoto, T. Inoue, and S. Namiki, "Disaggregated optical-layer switching for optically composable disaggregated computing [Invited]," in J. Opt. Commun. Netw., vol. 15, no. 1, pp. A11-A25 (2023).
https://doi.org/10.1364/jocn.471132 - R. Matsumoto, T. Inoue, R. Konoike, H. Matsuura, K. Suzuki, Y. Mori, K. Ikeda, S. Namiki, and K. Sato, "Scalable and Fast Optical Circuit Switch Based on Colorless Coherent Detection: Design Principle and Experimental Demonstration," in J. Lightwave Technol., vol. 39, no. 8, pp. 2263-2274 (2021).
https://doi.org/10.1109/JLT.2021.3050734 - R. Matsumoto, R. Konoike, K. Suzuki, H. Matsuura, K. Ikeda, T. Inoue, and S. Namiki, “Fully-Loaded Operation of 0.29-pJ/bit Wall-plug Efficiency, 81.9-Tb/s Throughput 32 × 32 Silicon Photonics Switch,” Proc. Optical Networking and Communication Conference & Exhibition (OFC), Tu6A.2, 2021.
https://doi.org/10.1364/OFC.2021.Tu6A.2 - T. Inoue, R. Matsumoto, and S. Namiki, "Learning-based digital back propagation to compensate for fiber nonlinearity considering self-phase and cross-phase modulation for wavelength-division multiplexed systems," in Opt. Express, vol. 30, 14851-14872 (2022).
https://doi.org/10.1364/OE.454841 - M. Pelusi, T. Inoue, and S. Namiki, "Enhanced Carrier to Noise Ratio by Brillouin Amplification for Optical Communications," in J. Lightwave Technol., vol. 38, no. 2, pp. 319-331 (2020).
https://doi.org/10.1109/JLT.2019.2948226 - A. Sobhanan, M. Pelusi, T. Inoue, D. Venkitesh, and S. Namiki, "Compensation of SOA-induced nonlinear phase distortions by optical phase conjugation," in Opt. Express, vol. 29, 12252-12265 (2021).
https://doi.org/10.1364/OE.416955